Laboratory for Electron Spectroscopy (LESSA)
A photoelectron spectroscopy and thin-film deposition facility supporting thin-film design, growth and analysis research programs.
RRID:SCR_022885
Training, experimental design and/or sample service.
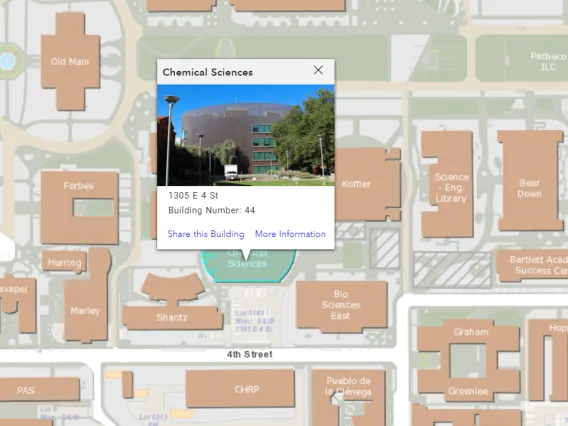
Visit Us!
Location:
Chemical Sciences Bldg. (CSB), Room 213
Hours:
Monday - Friday
9:00 AM to 5:00 PM
24/7 Access for Trained Users
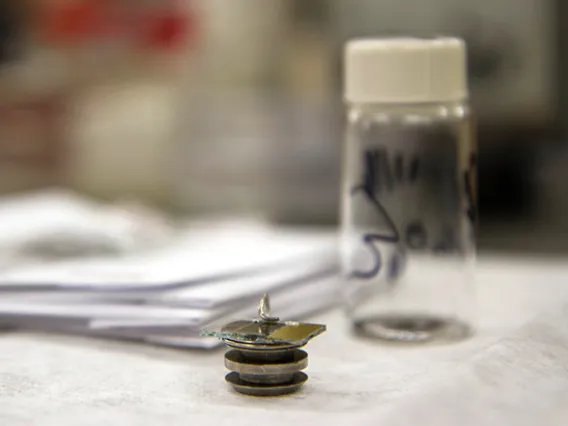
Services
LESSA uses Ultrahigh Vacuum X-ray and Ultraviolet Photoelectron Spectroscopy, (XPS and UPS, respectively) to examine the elemental composition (XPS) and valence electronic states(UPS) in the top 1-5 nm of the surface for any vacuum compatible material using a Kratos Axis 165 Ultra DLD Hybrid Ultrahigh Vacuum Photoelectron Spectrometer. The Photoelectric Effect is used to identify elements based on the calculated binding energy of a set of electrons to a nucleus, where each element has a different set of electron binding energies that are unique to the atom type and oxidation state. Ultraviolet Photoelectron Spectroscopy is used mainly to identify surface work functions and solid state Fermi/ionization energies for solid materials. The facility also has thin film deposition capabilities as well as controlled atmosphere sample introduction, if required. LESSA is a part of EPSILON.
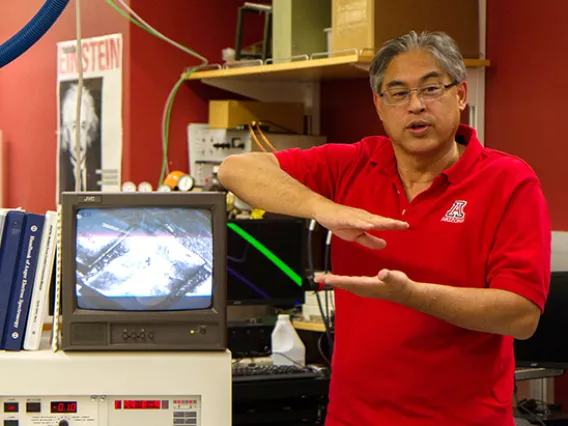
Education/Outreach
The LESSA Facility also provides educational outreach to groups interested in XPS and UPS with tours and lectures. This includes classes like CHM400a, CHM528 (undergraduate and graduate chemical instrumentation, respectively) and Engineering senior design projects. If you are interested in learning UHV surface analysis technique for training, tours or with school groups, contact the manager.
Facility Publications
Use the LESSA Facility RRID!
University of Arizona - UA CBC Laboratory for Electron Spectroscopy and Surface Analysis (LESSA) Facility, RRID:SCR_022885
91. Nicholas J. Wiebelhaus, Matthew A. Cranswick, Eric L. Klein, L. Tori Lockett, Dennis L. Lichtenberger,* and John H. Enemark. “Metal-Sulfur Valence Orbital Interaction Energies in Metal–Dithiolene Complexes: Determination of Charge and Overlap Interaction Energies by Comparison of Core and Valence Ionization Energy Shifts.” Inorg. Chem., 2011, submitted June, revision September.
90. Jaime A. Flores, José G. Andino, Nikolay P. Tsvetkov, Maren Pink, Robert J. Wolfe, Ashley R. Head, Dennis L. Lichtenberger, Joseph Massa, and Kenneth G. Caulton. “Assessment of the Electronic Structure of 2,2’-pyridylpyrrolides as Ligands.” Inorg. Chem., 2011, 50, 8121-8131.
89. Jinzhu Chen, Aaron K. Vannucci, Charles A. Mebi, Noriko Okumura, Susan C. Borowski, L. Tori Lockett, Matthew Swenson, Dennis L. Lichtenberger, Dennis H. Evans, and Richard S. Glass. “Catalysis of Electrochemical Reduction of Weak Acids to Produce H2: Role of O-H•••S Hydrogen Bonding.” Phosphorus, Sulfur and Silicon and the Related Elements, 2010, 186, 1288-1292. Invited paper.
88. Michael H. Palmer, Søren Vrønning Hoffmann, Nykola C. Jones, Ashley R. Head, Dennis L. Lichtenberger. “The Electronic States of 1,2,3-Triazole Studied by Vacuum UV Photoabsorption and UV Photoelectron Spectroscopy, and a Comparison with ab initio Configuration Interaction Methods.” J. Chem. Phys., 2011, 134, 084309 (13 pages)
87. Olga Lobanova Griffith, Adolphus G. Jones, John E. Anthony, and Dennis L. Lichtenberger. “Intermolecular Effects on the Hole States of Triisopropylsilylethynyl-Substituted Oligoacenes.” J. Phys. Chem. C, 2010, 114, 13838-13845.
86. Jinzhu Chen, Aaron K. Vannucci, Charles A. Mebi, Noriko Okumura, Susan C. Borowski, L. Tori Lockett, Dennis L. Lichtenberger, Dennis H. Evans and Richard S. Glass. “Synthesis of Diiron Hydrogenase Mimics Bearing Hydroquinone and Related Ligands. Electrochemical and Computational Studies of the Mechanism of Hydrogen Production and the Role of O–H•••S Hydrogen Bonding.” Organometallics, 2010, 29, 5330-5340. invited paper
85. Louis Y. Kuo, Curtis P. Smith, Dennis L. Lichtenberger and Ashley R. Head. “Phosphonothioate Hydrolysis through Selective P-S Bond Scission by Molybdenum Metallocenes” Main Group Chemistry, 2010, 9, 283-295.
84. Brandi M. Cossairt, Christopher C. Cummins, Ashley R. Head, Dennis L. Lichtenberger, Raphael J. F. Berger, Stuart A. Hayes, Norbert W. Mitzel, and Gang Wu. “On the Molecular and Electronic Structures of AsP3 and P4.” J. Am. Chem. Soc., 2010, 132, 8459-8465.
83. Mohammad K. Harb, Helmar Görls, Taka Sakamoto, Greg A. N. Felton, Dennis H. Evans, Richard S. Glass, Dennis L. Lichtenberger, Mohammad El-khateeb, and Wolfgang Weigand. “Synthesis and Characterization of [FeFe]-Hydrogenases Models with Bridging Moieties Containing (S, Se) and (S, Te).” Eur. J. Inorg. Chem., 2010, 25, 3976-3985.
82. Aaron K. Vannucci, Shihua Wang, Gary S. Nichol, Dennis L. Lichtenberger, Dennis H. Evans, and Richard S. Glass. “Electronic and Geometric Effects of Phosphatriazaadamantane Ligands on the Catalytic Activity of an [FeFe]-Hydrogenase Inspired Complex.” Dalton Trans., 2010, 12, 3050-3056.
81. Olga Lobanova Griffith, John E. Anthony, Adolphus G. Jones, and Dennis L. Lichtenberger. “Electronic Properties of Pentacene versus Triisopropylsilylethynyl-Substituted Pentacene: Environment-Dependent Effects of the Silyl Substituent.” J. Am. Chem. Soc., 2010, 132(2), 580-586.
80. Mohammad K. Harb, Ulf-Peter Apfel, Joachim Kübel, Helmar Görls, Greg A. N. Felton, Taka Sakamoto, Dennis H. Evans, Richard S. Glass, Dennis L. Lichtenberger, Mohammad El-khateeb and Wolfgang Weigand. “Preparation and Characterization of Diiron-dichalcogenolato Complexes Containing an Oxetane Ring: [FeFe]-Hydrogenase Models.” Organometallics, 2009, 28(23), 6666,6675.
79. Aaron K. Vannucci, Rae Ana Snyder, Nadine E. Gruhn, Dennis L. Lichtenberger and John H. Enemark. “New Insights into Solvolysis and Reorganization Energy from Gas-Phase, Electrochemical, and Theoretical Studies of Oxo-Tp*MoV Molecules” Inorg. Chem. 2009, 48(18), 8856-8862.
78. Harb, Mohammad K.; Windhager, Jochen; Daraosheh, Ahmad; Goerls, Helmar; Lockett, L. Tori; Okumura, Noriko; Evans, Dennis H.; Glass, Richard S.; Lichtenberger, Dennis L.; El-khateeb, Mohammad; Weigand, Wolfgang. “Phosphane- and Phosphite-Substituted Diiron Diselenolato Complexes as Models for [FeFe]-Hydrogenases.” Eur. J. Inorg. Chem., 2009, 23, 3414-3420.
77. Shentan Chen, Malcolm H. Chisholm, Ernest R. Davidson, Jason B. English and Dennis L. Lichtenberger. “Theoretical and Spectroscopic Investigations of the Bonding and Reactivity of (RO)3M≡N Molecules, where M = Cr, Mo, and W.” Inorg. Chem. 2009, 48(3), 828–837 (chosen for the cover article).
76. Mohammad K. Harb, Tobias Niksch, Jochen Windhager, Helmar Görls, Rudolf Holze, L. Tori Lockett, Noriko Okumura, Dennis H. Evans, Richard S. Glass, Dennis L. Lichtenberger, Mohammad El-khateeb and Wolfgang Weigand. “Synthesis and Characterization of Diiron Diselenato Complexes Including Iron Hydrogenase Models.” Organometallics, 2009, 28(4), 1039–1048.
75. Petro, Benjamin J.; Vannucci, Aaron K.; Lockett, L. Tori; Mebi, Charles; Kottani, Rudresha; Gruhn, Nadine E.; Nichol, Gary S.; Goodyer, Paul A. J.; Evans, Dennis H.; Glass, Richard S.; Lichtenberger, Dennis L. “Photoelectron spectroscopy of dithiolatodiironhexacarbonyl models for the active site of [Fe-Fe] hydrogenases: Insight into the reorganization energy of the "rotated" structure in the enzyme.” J. Mol. Struct. 2008, 890(1-3), 281-288.
74. Durivage, Jason C.; Gruhn, Nadine E.; Li, Bo; Dikarev, Evgeny V.; Lichtenberger, Dennis L. ”The Electronic Structure and Bonding of the First p-Block Paddlewheel Complex, Bi2(trifluoroacetate)4, and Comparison to d-Block Transition Metal Paddlewheel Complexes: A Photoelectron and Density Functional Theory Study.” J. Cluster Science 2008, 19(1), 275-294.
73. Anthony F. Cozzolino, Nadine E. Gruhn, Dennis L. Lichtenberger, and Ignacio Vargas-Baca. “Valence Electronic Structure of Benzo-2,1,3-chalcogenadiazoles Studied by Photoelectron Spectroscopy and Density Functional Theory.” Inorg. Chem., 2008, 47(14), 6220–6226.
72. Olga Lobanova Griffith, Nadine E. Gruhn, John E. Anthony, Balaji Purushothaman and Dennis L. Lichtenberger. “Electron Transfer Parameters of Triisopropylsilylethynyl-Substituted Oligoacenes.” J. Phys. Chem. C, 2008, 112 (51), 20518–20524.
71. Matthew A. Cranswick, Nadine E. Gruhn, John H. Enemark, and Dennis L. Lichtenberger. “Electronic Structure of the d1 Bent-metallocene Cp2VCl2: A Photoelectron and Density Functional Study.” J. Organomet. Chem., 2008, 693, 1621-1627.
70. Matthew A. Cranswick, Nadine E. Gruhn, Kristie R. Winfield, Nicolai Burzlaff, Wolfdieter A. Schenk, Dennis L. Lichtenberger. “Metal-sulfur dπ–pπ Buffering of the Oxidations of Metal-thiolate Complexes: Photoelectron Spectroscopy of (η5-C5H5)Fe(CO)2SR (SR = SCH3, StBu) and (η5-C5H5)Re(NO)(PR3)SCH3 (PR3 = PiPr3, PPh3).” Inorganica Chimica Acta, 2008, 36, 1122-1133.
69. Matthew A. Cranswick, Alice Dawson, J. Jon A. Cooney, Nadine E. Gruhn, Dennis L. Lichtenberger, John H. Enemark. “Photoelectron Spectroscopy and Electronic Structure Calculations of d1 Vanadocene Compounds with Chelated Dithiolate Ligands: Implications for Pyranopterin Mo/W Enzymes.” Inorg. Chem., 2007, 46, 10639-10646.
68. Greg A. N. Felton, Aaron K. Vannucci, Jinzhu Chen, Tori Moser, Noriko Okumura, Benjamin J. Petro, Uzma I. Zakai, Dennis H. Evans, Richard S. Glass and Dennis L. Lichtenberger. “Hydrogen Generation from Weak Acids: Electrochemical and Computational Studies of a Diiron Hydrogenase Mimic.” J. Am. Chem. Soc., 2007, 129(41), 12521-12530.
67. Nadine E. Gruhn and Dennis L. Lichtenberger, “Photoelectron Spectroscopy” in Encyclopedia of Inorganic Chemistry,” Robert A. Scott and Charles M. Lukehart, Ed. John Wiley & Sons Ltd., 2007.
66. Asha Rajapakshe, Rehan Basta, Atta M. Arif, Richard D. Ernst, and Dennis L. Lichtenberger. “Silyl Substitution Effects on Metal-Pentadienyl Bonding: Synthesis, Structure, Photoelectron Spectroscopy and Electronic Structure of a High Valent Half-Open Zirconocene.” Organometallics, 2007, 26(11), 2867-2871.
65. Block, E.; Glass, R. S.; Dikarev, E. V.; Gruhn, N. E.; Jin, J.; Li, B.; Lorance, E.; Zakai, U. I.; Zhang, S. Z. "Heteroatoms Galore. 1. Synthesis, Structure, and Photoelectron Spectroscopic Characterization of New, Mixed Sulfur-, Selenium- or Tellurium and Silicon- or Tin-containing Mesocycles", Heteroatom. Chem., 2007, 18, 509-515.
64. da Silva Filho, D. A.; Coropceanu, V.; Gruhn, N. E.; Fichou, D.; Bill, T. G.; Gierschner, J.; Cornil, J.; Brédas, J.-L. “Hole-Vibronic Coupling in Oligothiophenes: Impact of Backbone Torsional Flexibility on Relaxation Energies”, Phil. Trans. R. Soc. A, 2007, 365, 1435-1452.
63. Gruhn, N. E.; Macías-Ruvalcaba, N. A.; Evans, D. H. “Studies of Potential Inversion in an Extended Tetrathiafulvalene”, Langmuir, 2006, 22, 10683-10688.
62. Cotton, F. A.; Durivage, J. C.; Gruhn, N. E.; Lichtenberger, D. L.; Murillo, C. A.; Van Dorn, L. O.; Wilkinson, C. A. “Photoelectron Spectroscopy and DFT Calculations of Easily Ionized Quadruply Bonded Mo24+ Compounds and Their Bicyclic Guanidinate Precursors”, J. Phys. Chem. A, 2006, 110, 19793 - 19798.
61. Coropceanu, V.; Nakano, T.; Gruhn, N. E.; Kwon, O.; Yade, T.; Brédas, J.-L. “Probing Charge Transport in π-Stacked Fluorene-based Systems”, J. Phys. Chem. B, 2006, 110, 9482-9487.
60. Rajapakshe, A.; Paz-Sandoval, M. A.; Gutierrez, J. A.; Navarro-Clemente, J. E.; Saavedra, P. A., Gruhn, N. E.; Lichtenberger, D. L. “Heteropentadienyl Analogs of Half-Open Ruthenocenes: Metal-Ligand Interactions and Electronic Structure Perturbations”, Organometallics, 2006, 25, 1914-1923.
59. Gruhn, N. E.; Macías-Ruvalcaba, N. A.; Evans, D. H. “Studies of the Inner Reorganization Energies of the Cation Radicals of 1,4-bis(Dimethylamino)benzene, 9,10-bis(Dimethylamino)anthracene and 3,6-bis(Dimethylamino)durene by Photoelectron Spectroscopy and Reinterpretation of the Mechanism of the Electrochemical Oxidation of the Parent Diamines”, J. Phys. Chem. A, 2006, 110, 5650-5655.
58. Glass, R. S.; Block, E.; Lorance, E.; Zakai, U. I.; Gruhn, N. E.; Jin, J.; Zhang, S.-Z. "The Si Si Effect on Ionization of beta Disilanyl Sulfides and Selenides", J. Am. Chem. Soc., 2006, in press.
57. Cotton, F. A.; Donahue, J. P.; Gruhn, N. E.; Lichtenberger, D. L.; Murillo, C. A.; Timmons, D. J.; Van Dorn, L. O.; Villagrán, D.; Wang, X. "Facilitating Access to the Most Easily Ionized Molecule: an Improved Synthesis of the Key Intermediate, W2(hpp)4Cl2, and Related Compounds", Inorg. Chem., 2006, 45, 201-213.
56. Coropceanu, V.; Kwon, O.; Wex, B.; Kaafarani, B. R.; Gruhn, N. E.; Durivage, J. C.; Neckers, D. C.; Bredas, J.-L. "Vibronic Coupling in Organic Semiconductors: The Case of Fused Benzene-Thiophene Structure" Chem. Eur. J. 2006, 4, 2073-2080.
55. Glass, R. S.; Gruhn, N. E.; Lorance, E.; Singh, M. S.; Stessman, N. Y. T.; Zakai, U. I. "Synthesis, Gas-Phase Photoelectron Spectroscopic, and Theoretical Studies of Stannylated Dinuclear Iron Dithiolates", Inorg. Chem. 2005, 44, 5728-5737.
54. Lambert, C.; Risko, C.; Coropceanu, V.; Schelter, J.; Amthor, S.; Gruhn, N. E.; Durivage, J. C.; Bredas, J. L. "Electronic Coupling in Tetraanisylarylenediamine Mixed Valence Systems: The Interplay between Bridge Energy and Geometric Factors" J. Am. Chem. Soc. 2005, 127, 8508-8516.
53. Cunico, R. F.; Motta, A. R. "Addition of Carbamoylsilanes to Electrophilically Substituted Alkenes. Preparation of β-Functionalized Tertiary Amides", Org. Lett., 2005, 7, 771-774.
52. Cooney, J. J. A.; Cranswick, M. A.; Gruhn, N. E.; Joshi, H. K.; Enemark, J. H. "Electronic Structure of Bent Titanocene Complexes with Chelated Dithiolate Ligands", Inorg. Chem., 2004, in press.
51. Rajapakshe, A.; Gruhn, N. E.; Lichtenberger, D. L.; Basta, R.; Arif, A. M.; Ernst, R. D. "Pentadienyls vs Cyclopentadienyls and Reversal of Metal-Ligand Bonding Affinity with Metal Oxidation State: Synthesis, Molecular Structures, and Electronic Structures of High-Valent Zirconium Pentadienyl Complexes", J. Am. Chem. Soc., 2004, 126, 14105-14116.
50. Kwon, O.; Coropceanu, V.; Gruhn, N. E.; Durivage, J. C.; Laquindanum, J. G.; Katz, H. E.; Cornil, J.; Brédas, J. L. "Characterization of the Molecular Parameters Determining Charge Transport in Anthradithiophene", J. Chem. Phys., 2004, 120, 8186-8194.
49. Coropceanu, V.; Gruhn, N. E.; Barlow, S.; Lambert, C.; Durivage, J. C.; Bill, T. G.; Nöll, S. G.; Marder, S. R.; Brédas, J. L. "Electronic Couplings in Organic Mixed-Valence Compounds: The Contribution of Photoelectron Spectroscopy", J. Am. Chem. Soc., 2004, 126, 2727-2731.
48. Joshi, H. K.; Arvin, M. E.; Durivage, J. C.; Gruhn, N. E.; Carducci, M. D.; Westcott, B. L.; Lichtenberger, D. L.; Enemark, J. H. "Photoelectron Spectra of Potassium Salts of Hydrotris(1-pyrazolyl)borates: Electronic Structure of the Electron Withdrawing Scorpionates Tp(CF3)2, Tp*Cl and Comparison to Tp* and Tp", Polyhedron, 2004, 23, 429-438.
47. Amashukeli, X.; Gruhn, N. E.; Lichtenberger, D. L.; Winkler, J. R.; Gray, H. B. "Inner-Sphere Electron-Transfer Reorganization Energies of Zinc Porphyrins", J. Am. Chem. Soc., 2004, in press.
46. Westcott, B. L.; Crundwell, G.; Burkholder, T. R.; Michelsen, L. J.; Gardner, C. B.; Gruhn, N. E.; Hunter, A. B.; Miner, P.; Zeller, M. "The Molecular and Electronic Structure of N,N'-Ethylenebis(acetyl-acetonylideiminato)oxovanadium(IV) and the Electronic Structure of its Thio Analogue", J. Electron Spectrosc. Relat. Phenom., 2004, 135, 1-5.
45. Lorance, E. D.; Glass, R. S.; Block, E.; Li, X. "Synthesis, Electrochemistry, and Gas-Phase Photoelectron Spectroscopic and Theoretical Studies of 3,6-Bis(perfluoroalkyl)-1,2-dithiins", J. Org. Chem., 2003, 68, 8110-8114.
44. Alloway, D. M.; Hofmann, M.; Smith, D. L.; Gruhn, N. E.; Graham, A. L.; Colorado, R., Jr.; Wysocki, V. H.; Lee, T. R.; Lee, P. A.; Armstrong, N. R. "Interface Dipoles Arising from Self-Assembled Monolayers on Gold: UV-Photoemission Studies of Alkanethiols and Semi-fluorinated Alkanethiols", J. Phys. Chem. B, 2003, 107, 11690-11699.
43. Joshi, H. K.; Cooney, J. J. A.; Inscore, F. E.; Gruhn, N. E.; Lichtenberger, D. L.; Enemark, J. H. "Investigation of Metal-Dithiolate Fold Angle Effects by Gas Phase Photoelectron Spectroscopy and Density Functional Calculations: Implications for Molybdenum Enzymes", Proc. Natl. Acad. Sci. USA, 2003, 100, 3719-3724.
42. Rathore, R.; Abdelwahed, S. H.; Guzei, I. A. "Synthesis, Structure, and Evaluation of the Effect of Multiple Stacking on the Electron-Donor Properties of π-Stacked Polyfluorenes", J. Am. Chem. Soc., 2003, 125, 8712-8713.
41. Lichtenberger, D. L. "Electron Distribution, Bonding, and J(Si-H) NMR Coupling Constant in (η5-C5H5)(CO)2MnHSiCl3: The Molecular Orbital View", Organometallics, 2003, 22, 1599-1602.
40. Cotton, F. A.; Gruhn, N. E.; Gu, J.; Huang, P.; Lichtenberger, D. L.; Murillo, C. A.; Van Dorn, L. O.; Wilkinson, C. C. "Closed-shell Molecules that Ionize More Readily than Cesium", Science, 2002, 298, 1971-1974.
39. Joshi, H. K.; Inscore, F. E.; Schirlin, J. T.; Dhawan, I. K.; Carducci, M. D.; Bill, T. G.; Enemark, J. H. "Six-coordinate molybdenum nitrosyls with a single ene-1,2-dithiolate ligand", Inorg. Chim. Acta, 2002, 337, 275-286.
38. Lichtenberger, D. L.; Fan, H.-J.; Gruhn, N. E. "Ligand-Mediated Metal-Metal Interactions and Localized versus Delocalized Mixed-Valence Cation States of Biferrocene and Bis(μ-fulvalenediyl)diiron Characterized in the Gas Phase by Valence Photoelectron Spectroscopy", J. Organomet. Chem., 2003, 666, 75-85.
37. Gruhn, N. E.; Lichtenberger, D. L. "Photoelectron Spectroscopy of Inorganic Molecules", in The Encyclopedia of Mass Spectrometry, Vol. 1, P. B. Armentrout, Volume Editor, Elsevier, 2003, 818-833.
36. Padden Metzker, J. K.; Lichtenberger, D. L.; Lee, P. E. "Valence Photoelectron Spectroscopy of Non-volatile Organometallic Molecules", in Thin Films: Preparation, Characterization, Applications, Soriaga, M. P.; Stickney, J.; Bottomley, L. A.; Kim, Y.-G., Editors, Kluwer Academic/Plenum Publishers, 2002, 301-307.
35. Coropceanu, V.; Malagoli, M.; da Silva Filho, D. A.; Gruhn, N. E.; Bill, T. G.; Brédas, J. L. "Hole- and electron-vibrational couplings in oligoacene crystals: Intramolecular contributions", Phys. Rev. Lett., 2002, 89, 275503.
34. Padden Metzker, J. K.; Gruhn, N. E.; Lichtenberger, D. L. "Transferability of the Valence Ionization Intensities of Chemical Functional Groups Between Molecules", J. Phys. Chem. A, 2002, 106, 9999-10009.
33. Gruhn, N. E.; Michelsen, L. J.; Westcott, B. L. "Photoelectron Spectroscopy of Bis(2,4-Pentanedione)-Oxovanadium(IV) [VO(acac)2] and Derivatives: Substituent Effects on the 2,4-Pentanedione Donor", Inorg. Chem., 2002, 41, 5907-5911.
32. Lichtenberger, D. L.; Gruhn, N. E.; Rai-Chaudhuri, A.; Renshaw, S. K.; Gladysz, J. A.; Seyler, J.; Igau, A. "How do the Electronic Structures of Low-Symmetry Metal-Hydride and -Alkyl Complexes Compare? Photoelectron Spectroscopy and Computational Studies of (η5-C5R5)Re(NO)(L)R' [L = CO, P(C6H5)3; R, R' = H, CH3)]", Organometallics, 2002, 21, 5494-5504.
31. Pascal, L.; Van den Eynde, J. J.; Van Haverbeke, Y.; Dubois, P.; Michel, A.; Rant, U.; Zojer, E.; Leising, G.; Van Dorn, L. O.; Gruhn, N. E.; Cornil, J.; Brédas, J. L. "Synthesis and Characterization of Novel para- and meta-Phenylenevinylene Derivatives: Fine Tuning of the Electronic and Optical Properties of Conjugated Materials", J. Phys. Chem. B, 2002, 106, 6442-6450.
30. Gruhn, N. E.; Silva Filho, D. A.; Bill, T. G.; Malagoli, M.; Coropceanu, V.; Kahn, A.; Brédas, J. L. "The Vibrational Reorganization Energy in Pentacene: Molecular Influences on Charge Transport", J. Am. Chem. Soc., 2002, 124, 7918-7919.
29. Graff, J. N.; McElhaney, A. E.; Basu, P.; Gruhn, N. E.; Chang, C.-C. J.; Enemark, J. H. "Electrochemistry and Photoelectron Spectroscopy of Oxomolybdenum(V) Complexes with Phenoxide Ligands: Effect of Para Substituents on Redox Potentials, Heterogeneous Electron Transfer Rates, and Ionization Energy", Inorg. Chem., 2002, 41, 2642-2647.
28. Amashukeli, X.; Winkler, J. R.; Gray, H. B.; Gruhn, N. E.; Lichtenberger, D. L. "Electron-Transfer Reorganization Energies of Isolated Organic Molecules", J. Phys. Chem. A, 2002, 106, 7593-7598.
27. Lichtenberger, D. L.; Gruhn, N. E.; Rai-Chaudhuri, A.; Renshaw, S. K.; Gladysz, J. A.; Jaio, H.; Seyler, J.; Igau, A. "Vibrational Progressions in the Valence Ionizations of Transition Metal Hydrides: Evaluation of Metal-Hydride Bonding and Vibrations in (η5-C5R5)Re(NO)(CO)H [R = H, CH3]", J. Am. Chem. Soc., 2002, 124, 1417-1423.
26. Slattery, D. K.; Linkous, C. A.; Gruhn, N. E.; Baum, J. C. "Semi-Empirical MO and Voltammetric Estimation of Ionization Potentials of Organic Pigments. Comparison to Gas-Phase Ultraviolet Photoelectron Spectroscopy", Dyes and Pigments, 2001, 49, 21-27.
25. Cornil, J.; Gruhn, N. E.; dos Santos, D. A.; Malagoli, M.; Lee, P. A.; Barlow, S.; Thayumanavan, S.; Marder, S. R.; Armstrong, N. R.; Brédas, J. L. "Joint Experimental and Theoretical Characterization of the Electronic Structure of 4,4'-bis(N-m-tolyl-N-phenylamino)biphenyl (TPD) and Substituted Derivatives", J. Phys. Chem. A, 2001, 105, 5206-5211.
24. Schlettwein, D.; Hesse, K.; Gruhn, N. E.; Lee, P. A.; Nebesny, K. W.; Armstrong, N. R. "Electronic Energy Levels in Individual Molecules, Thin Films and Organic Heterojunctions of Substituted Phthalocyanines", J. Phys. Chem. B, 2001, 105, 4791-4800.
23. Slattery, D. K.; Linkous, C. A.; Gruhn, N. E. "Organic Pigments as Photocatalytic Agents in a Solar Water-Splitting Scheme", Hydrogen Energy Progress XIII : Proceedings of the 13th World Hydrogen Energy Conference, June 12-15, 2000, Beijing, China, T.N. Veziroglu and Z.Q. Mao, Editors.
22. "Synthesis, Properties, Oxidation, and Electrochemistry of 1,2-Dichalcogenins", E. Block, M. Birringer, R. DeOrazio, J. Fabian, R. S. Glass, C. Guo, C. He, E. Lorance, Q. Qian, T. B. Schroeder, Z. Shan, M. Thiruvazhi, G. S. Wilson, and X. Zhang, J. Am. Chem. Soc. 2000, 122, 5052-5064.
21. Glass, R. S.; Gruhn, N. E.; Lichtenberger, D. L.; Lorance, E. D.; Pollard, J. R.; Birringer, M.; Block, E.; DeOrazio, R.; He, C.; Shan, Z.; Zhang, X. "Gas-Phase Photoelectron Spectroscopic and Theoretical Studies of 1,2-Dichalcogenins. Ionization Energies, Orbital Assignments, and an Explanation of Their Color", J. Am. Chem. Soc., 2000, 122, 5065-5074.
20. Helton, M. E.; Gruhn, N. E.; McNaughton, R.; Kirk, M. L. "Control of Oxo-Molybdenum Reduction and Ionization Potentials by Dithiolate Donors", Inorg. Chem., 2000, 39, 2273-2278.
19. Lichtenberger, D. L.; Fan, H.-J.; Gruhn, N. E.; Bitterwolf, T. E.; Gallagher, S. "Electronic Structure and Photoelectron Spectroscopic Studies on CpM(CO)4 (M=V, Nb, Ta)" Organometallics, 2000, 19, 2012-2021.
18. Smith, K. J.; Ondracek, A. L.; Gruhn, N. E.; Lichtenberger, D. L.; Fanwick, P. E.; Walton, R. A. "A comparative study of the isomers of ReOCl3(PMe3)2 and ReOCl3(PEt3)2. The isolation and characterization of ReH7(PR3)2 and ReO(OEt)Cl2(PR3)2 (R = Me or Et) and the photoelectron spectrum of ReH7(PMe3)2", Inorg. Chim. Acta 2000, 300-302, 23-31.
17. Westcott, B. L.; Gruhn, N. E.; Michelsen, L. J.; Lichtenberger, D. L. "Experimental Observation of Non-Aufbau Behavior: Photoelectron Spectra of Vanadyloctaethylporphyrinate and Vanadylphthalocyanine", J. Am. Chem. Soc. 2000, 122, 8083-8084.
16. Asirvatham, V. S.; Gruhn, N. E.; Lichtenberger, D. L.; Ashby, M. T. "Electronic Factors for Protonation of an Organometallic Molecule. A Photoelectron Spectroscopy and Electron Paramagnetic Resonance Study of [η5-C6H6)Mo(TRIPOD)]0/+", Organometallics, 2000, 19, 2215-2227.
15. Slattery, D. K.; Linkous, C. A.; Gruhn, N. E. "Photocatalytic Water-Splitting Using Organic Pigments as Semiconductors", Polymer Preprints, 2000, 41(1), 866-867.
14. Gruhn, N. E.; Lichtenberger, D. L.; Ogura, H.; Walker, F. A. "Reevaluation of the Gas-Phase Valence Photoelectron Spectra of Octaethylporphyrin and Tetraphenylporphyrin", Inorg. Chem. 1999, 38, 4023-4027.
13. Lichtenberger, D. L.; Pollard, J. R.; Lynn, M. A.; Cotton, F. A.; Feng, X. "Metal-Metal Bonding in Rh2(O2CCF3)4. Extensive Metal-Ligand Orbital Mixing Promoted by Filled Fluorine Orbitals", J. Am. Chem. Soc. 2000, 122, 3182-3190.
12. Lichtenberger, D. L.; Lynn, M. A.; Chisholm, M. H. "Quadruple Metal-Metal Bonds with Strong Donor Ligands. Ultraviolet Photolectron Spectroscopy of M2(form)4 (M = Cr, Mo, W; form = N,N'-diphenylformamidinate)", J. Am. Chem. Soc. 1999, 121, 12167-12176.
11. Cornil, J.; Vanderdonckt, S.; Lazzaroni, R.; dos Santos, D. A.; Thys, G.; Geise, H. J.; Yu, L.-M.; Lögdlund, M.; Salaneck, W. R.; Gruhn, N. E.; Lichtenberger, D. L.; Lee, P. A.; Armstrong, N. R.; Brédas, J. L. "Valence Electron Structure of π-Conjugated Materials: Simulation of the Ultraviolet Photoelectron Spectra with Semiempirical Hartree-Fock Approaches", Chem. Mat. 1999, 11, 2436-2443.
10. Gruhn, N. E.; Lichtenberger, D. L. "Characterization of the Electronic Structure of Transition Metal Carbonyls and Metallocenes", in Inorganic Electronic Structure and Spectroscopy, Vol. 2, A. B. P. Lever and E. I. Solomon, Eds., John Wiley and Sons, 1999, pp 533-574.
9. Anderson, J. D.; McDonald, E. M.; Lee, P. A.; Anderson, M. L.; Ritchie, E. L.; Hall, H. K.; Hopkins, T.; Mash, E. A.; Wang, J.; Padias, A.; Thayumanavan, S.; Barlow, S.; Marder, S. R.; Jabbour, G. E.; Shaheen, S.; Kippelen, B.; Peyghambarian, N.; Wightman, R. M.; Armstrong, N. R. "Electrochemistry and Electrogenerated Chemiluminescence Processes of the Components of Aluminum Quinolate/Triarylamine, and Related Organic Light-Emitting Diodes", J. Am. Chem. Soc. 1998, 120, 9646-9655.
8. Westcott, B. L.; Gruhn, N. E.; Enemark, J. H. "Evaluation of Molybdenum-Sulfur Interactions in Molybdoenzyme Model Complexes by Gas-Phase Photoelectron Spectroscopy: The "Electronic Buffer" Effect", J. Am. Chem. Soc. 1998, 120, 3382-3386.
7. Budzichowski, T. A.; Chisholm, M. H.; Tiedtke, D. B.; Gruhn, N. E.; Lichtenberger, D. L. "Preparation, Characterization and Electronic Structure of W2(NMe2)2(ORf)4, where Rf = CMe2CF3, CMe(CF3)2 and C(CF3)3, as Deduced by Photoelectron Spectroscopic Studies and the Single Crystal X-ray Structure of Rf = CMe(CF3)2", Polyhedron, 1998, 17, 705-711.
6. Westcott B. L.; Enemark, J. H. "Formal Oxidation States vs. pi-effects in Isostructural Low-Symmetry {MoNO}+3 and {MoO}+3 Complexes", Inorg. Chem., 1997, 36, 5404-5405.
5. Glass, R. S.; Pollard, J. R.; Schroeder, B.; Lichtenberger, D. L.; Block, E.; DeOrazio, R.; Guo, C.; Thiruvazhi, M. "Spectroscopic, Theoretical, and Electrochemical Studies of 1,2-Dithiins", Phosphorus, Sulfur, and Silicon, 1997, 120/121, 439-440.
4. Block, E.; Glass, R. S.; DeOrazio, R.; Lichtenberger, D. L.; Pollard, J. R.; Russell, E. E.; Schroeder, B.; Thiruvazhi M.; Toscano, P. J. "Significant Intramolecular Sulfur-Sulfur Interactions in cis- and trans-2,3-Dimethyl-5,6-dithiabicyclo[2.1.1]hexane", SYNLETT, 1997, 5, 525-528.
3. Lichtenberger, D. L.; Elkadi, Y.; Gruhn, N. E.; Hughes, R. P.; Curnow O. J.; Zheng, X. "Electronic Structure Perturbations of Substituted Ruthenocenes: The First Photoelectron Spectra of Perchloro- and Perfluorocyclopentadienyl Complexes", Organometallics, 1997, 16, 5209-5217.
2. Drouin, B. J.; Gruhn, N. E.; Madden, J. F.; Kukolich, S. G.; Barfield, M.; Glass, R. S. "Gas-Phase Conformational Analysis of 1,4,7-Trithiacyclononane", J. Phys. Chem. A, 1997, 101, 9180-9184.
1. Lichtenberger, D. L.; Gruhn N. E.; Renshaw, S. K. "Relative Bonding Capabilities of Molecules to Metals as Measured by Gas-Phase Photoelectron Spectroscopy", J. Mol. Struct. 1997, 405, 79-86.