Jeanne E. Pemberton*
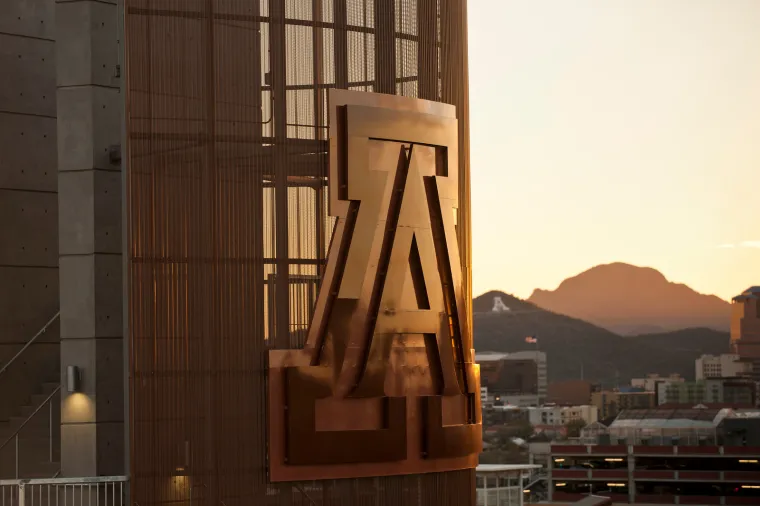
Chemical Sciences Building, Room 302
Degrees and Appointments
- Ph.D. University of North Carolina, Chapel Hill
- B.S. University of Delaware (Chemistry)
- B.A. University of Delaware (Biology)
Specialties: Chemical Reaction Dynamics/Kinetics/Interactions​, Energy Science, Instrument Development, Materials and Polymer Chemistry, Spectroscopy/Molecular Structure, Surface and Solid State, Synthesis/Synthetic Methods Development
Research Description
The Chemistry of Organic Semiconductors and their Interfaces in Molecular Electronic & Photonic Devices: The interfacial regions between phases are sites of critical importance in many relevant processes and technologies. Despite decades of intense study, our understanding of the chemistry of interfacial and surface processes at the molecular level is still only modestly developed. Thus, the development of adequate tools with which to study surface and interfacial chemistry and elucidation of the molecular details of such complex chemistry represent two of the most exciting frontiers of modern measurement science. Our research seeks to develop an understanding of such chemistry in several technologically important areas including organic semiconductor-based devices.