Professor
Degrees and Appointments
- M.S. 1986, Northern Arizona University
- Ph.D. 1991, University of California, Irvine
- Technical Staff 1991-2003, Sandia National Labs
- Technical Staff 2003-2005, Los Alamos National LabsAdjunct Professor 2001-2005, University of New Mexico
Field of Study: Organic Chemistry
Awards and Honors
- NNSA Defense Programs Award of Excellence for Significant Contributions to the Stockpile Stewardship, 2002
- Distinguished Member of Technical Staff, Sandia National Laboratories, 2001-2003
- Sandia National Labs Employee Recognition Team Awards, 1999
Research Specialties: Energy Science, Instrument Development, Materials and Polymer Chemistry, Surface and Solid State, Synthesis/Synthetic Methods Development
Research
Fuel Cell Electrolyte Membranes
The goal of our fuel cell membrane research is a cheap, easily processed, oxidatively stable, proton conducting membrane with high glass transition temperature and tailorable gas permeabilities. We have recently (with C. Cornelius & Cy Fujimoto at Sandia National Labs) developed a class of sulfonated polyarylenes (Figure 1) as polymeric electrolytes with excellent proton conductivity, thermal stability, mechanical properties and chemical stability.1 The polymer electrolytes were prepared by a Diels-Alder polymerization followed by sulfonation. Hydrogen-oxygen fuel cells built with these membranes out-performed fuel cells built with commercially available membranes. Future efforts will focus on new, more economical syntheses of the Diels-Alder polymers, using regiospecific chemistries to create tailored polymer morphologies, and templating strategies to control membrane morphology and functionality.
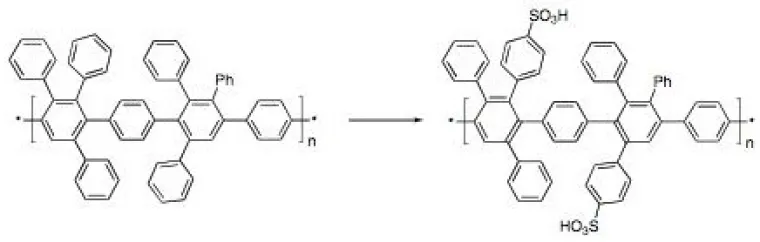
Figure 1. Sulfonation of polyarylenes for fuel cells.1
Environmentally Friendly Materials
This topic encompasses a diverse group of projects that all are directed at reducing the environmental impact of chemical processes and applications. Included in this group are highly parallel and combinatorial approaches to screening catalysts for hydrodesulfurization processing of low sulfur fuel (Figure 2),2 solvent-free sol-gel/encapsulation systems (Figure 3),3 self-developing photoresists,4 and improved metal scavengers from recovering precious metals. This group of environmentally friendly materials also includes a class of removable encapsulants (Figure 4) based on the Diels-Alder reaction that were originally developed to study thermodynamically controlled sol-gel architectures (with D. Wheeler at Sandia National Labs).5
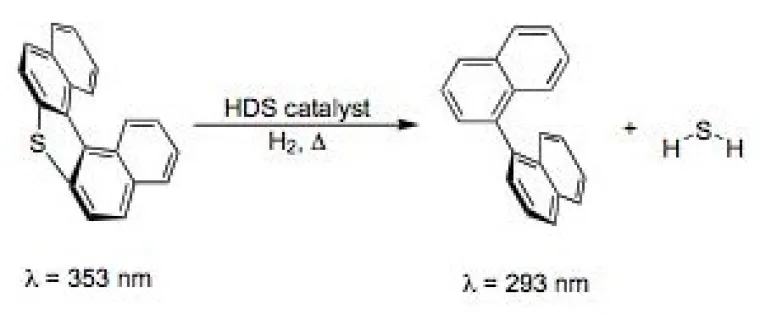
Figure 2. Thiophene dye for screening hydrodesulfurization catalysts undergoes blue shift in fluorescence with loss of sulfur.2
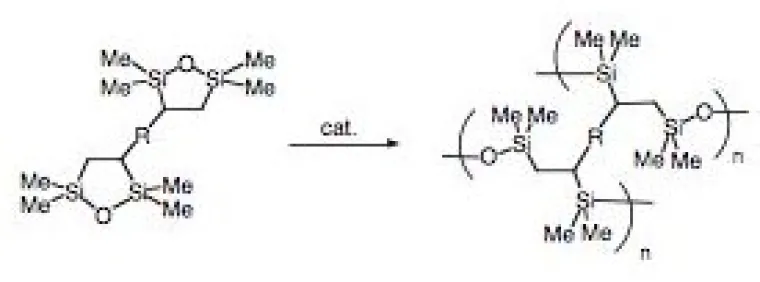
Figure 3. Solvent-free non-shrinking sol-gels based on ring opening polymerizations of disilacyclopentane groups produce little volatile organic contaminants (VOC’s).3
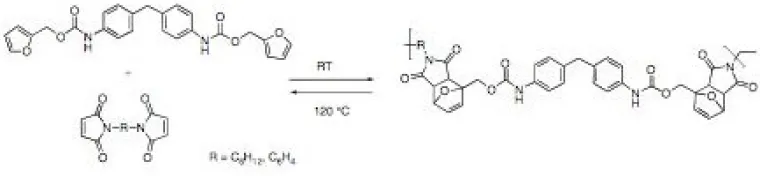
Figure 4. Polymers formed and depolymerized based on the thermoreversible Diels-Alder reaction.5
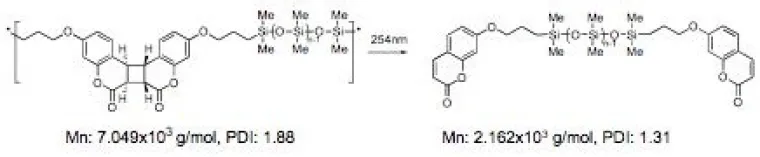
Figure 5. Coumarin dimers as photo-cleavable weak-links in polymeric photoresists5
The success of the thermally reversible polymers has led to the development of reversible photoresists (with K. J. Shea at UC Irvine) based on polymers with coumarin dimers groups as photocleavable weak-links (Figure 5).
Gas Separation Membranes
Membranes provide an energy efficient technology for separating gases and petrochemicals. Preliminary investigations of bridged polysilsesquioxane membranes (with C. Cornelius at Sandia National Labs) revealed extremely high selectivity for hydrogen over carbon dioxide or methane (Figure 6). However, the origins of this selectivity in these amorphous, intractable sol-gel materials are not well understood. Furthermore, sol-gel processing of membranes affords low yields of defect free membranes with the majority of the materials and membranes being discarded. Our efforts are focused on characterizing these materials using 2-D solid state NMR, porosimetry, electron microscopy, and permeability studies and developing new methods for preparing defect free membranes with minimal waste. One fundamental question being investigated is the relationship between hybrid network architectures and whether or not the thin film membranes retain porosity or not.
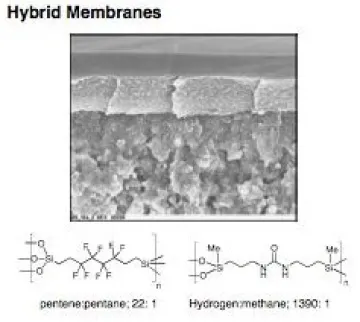
Figure 6. Sol-gel processing of bridged polysilsesquioxanes can be used to fabricate thin membranes with potential applications for hydrogen separation and for olefin-paraffin separations.
We are also evaluating an additional number of polymers and hybrid materials as membranes for gas separations. One promising candidate for high flux carbon dioxide selective membranes is the hybrid organic-inorganic based on Nylon-1 templated pores that builds on our previously developed pore templating strategies (Figure 7).6 We have also recently shown that polyarylenes have high gas permeabilities.
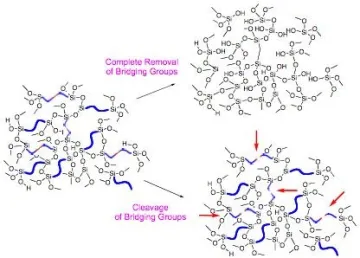
Figure 7. Bridging groups in hybrid sol-gel processed materials serve as convenient templates that can be removed completely by oxidation or partially through chemical reactions.6
Materials for Sensors
Our interests include the development of more selective, responsive materials for surface acoustic wave (SAW) sensors, scintillation materials for radiation detection, bio-material based sensors, and smart materials to report mechanical damage or physical changes in an engineering polymer or in a glass. Hybrid materials are a versatile platform for engineering the physical properties (mechanical strength, glass transition temperature, porosity) and chemical functionality to meet the needs of a broad range of sensors. One promising new method for generating lithographically defined, porous thin films with tailored thermo-mechanical properties is the base-catalyzed disproportionation of hydridosiloxanes (Figure 8; with K. Rahimian, Sandia Labs).7

Figure 8. Catalytic amounts of base cause the disproportionation of hydridosiloxanes to afford polysilsesquioxane foams.7
Aerogels
Our research on aerogels (with Kimberley DeFriend at Los Alamos National Laboratories) is primarily focused on the synthesis of mechanically robust, low-density materials with controlled porosity and composition (Figure 9). This effort includes investigations into the fundamental sol-gel chemistry of silsesquioxanes,8 the influence of substituents9 and reaction conditions10 on gelation, effects of aging gels on porosity, and use of chemical vapor deposition to modify and strengthen aerogels.
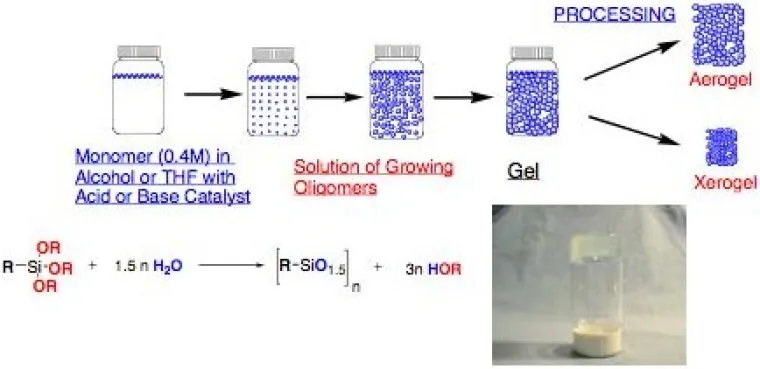
Figure 9. Sol-gel processing of alkoxysilanes affords gels that can be processed to afford xerogels or aerogels.
Fujimoto, C. H.; Hickner, M. A.; Cornelius, C. J.; Loy, D .A. Macromolecules (2005) in Press.
Staiger, C. L.; Loy, D. A.; Jamison, G. M.; Schneider, D. A.; Cornelius, C. J. J. Am. Chem. Soc. (2003) 125(33), 9920.
Loy, D. A.; Rahimian, K.; Samara, M. Angew. Chem., Int. Ed. (1999) 38(4), 555.
Beach, J. V.; Loy, D. A.; Hsiao, Y.-L.; Waymouth, R. M. ACS Symp. Ser. (1995), 614 (Microelectronics Technology), 355.
Mcelhanon, J. R.; Russick, E. M.; Wheeler, D. R.; Loy, D. A.; Aubert, J. H. Journal of Appl. Polym. Sci. (2002), 85(7), 1496.
Loy, D. A.; Shea, K. J.; Buss, R. J.; Assink, R. A. ACS Symp. Ser. (1994), 572 (Inorganic and Organometallic Polymers II), 122.
Rahimian, K.; Assink, R. A.; Lang, D. P.; Loy, D. A. Mater. Res. Soc. Symp. Proc. (2001) 628 (Organic/Inorganic Hybrid Materials), CC6.34.1-CC6.34.6.
Shea, K.J.; Loy, D. A., Chem. Mater. (2001), 13(10), 3306.
Loy, D. A. Chem. Mater. (2000) 12, 3624.
Loy, D. A.; Mather, B.; Straumanis, A. R.; Baugher, C.; Schneider, D. A.; Sanchez, A.; Shea, K. J. Chem. Mater. (2004), 16(11), 2041-2043.